30 Visual System: Central Processing
In this chapter we will learn about how the information from the retina is processed centrally within the brain.
Visual Fields
Before learning the pathway that visual information takes from the retina to the cortex, it is necessary to understand how the retina views the world around us. The full visual field includes everything we can see without moving our head or eyes.
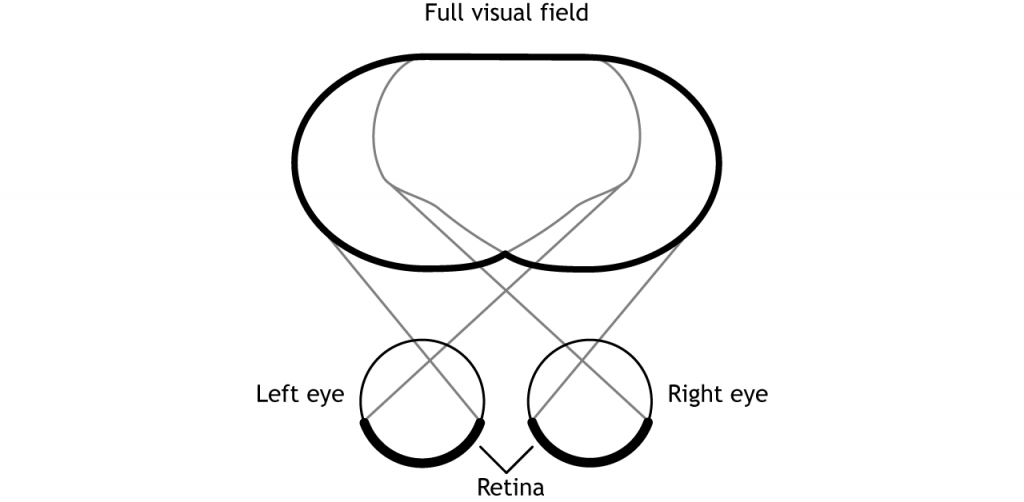
The full visual field can be divided in a few ways. Each individual eye is capable of seeing a portion of, but not the entire, visual field.
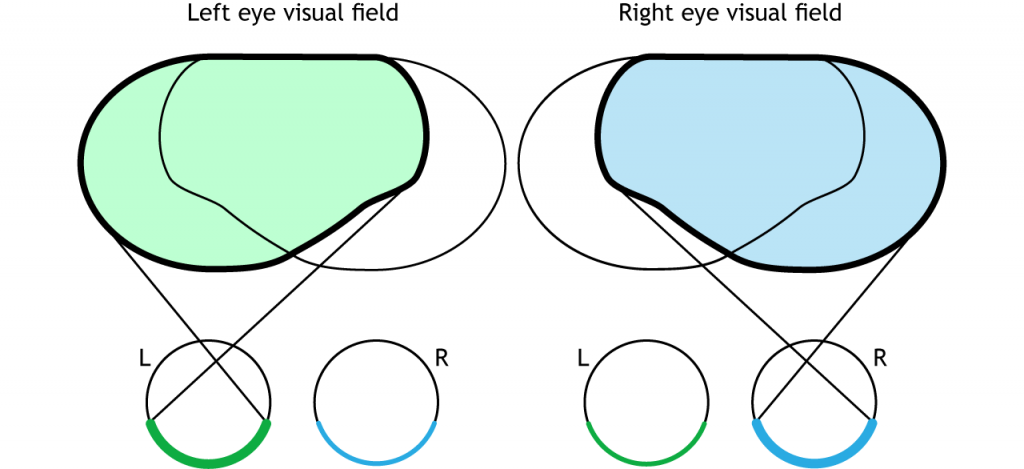
The full visual field can also be divided into the right and left hemifields. The hemifields range from the most peripheral point to the center point, splitting the full visual field into two equal regions. Both eyes are involved in viewing each hemifield. The fovea separates the retina into two sections: the nasal retina and the temporal retina. The nasal retina is the medial portion that is located toward the nose. The temporal retina is the lateral portion that is located toward the temples and temporal lobe. The nasal retina from one eye along with the temporal retina from the other eye are able to view an entire hemifield.
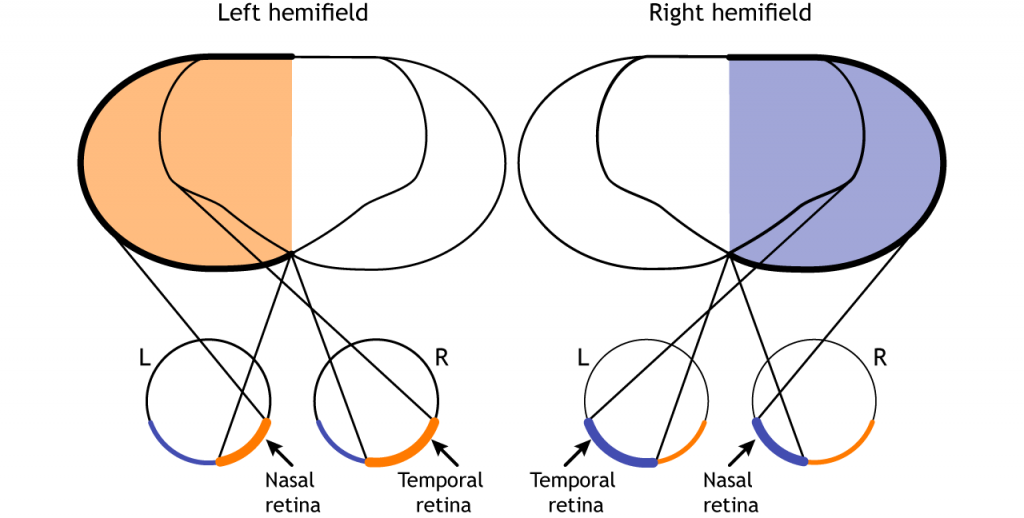
Finally, the full visual field can be separated into monocular and binocular regions. Each monocular field is visual space that can only be viewed by one eye. The binocular region is visual space that can be viewed by both eyes.
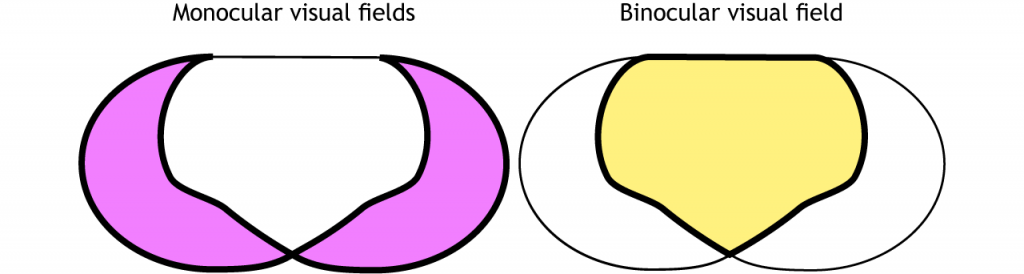
Pathway to Brain
Visual information from each eye leaves the retina via the ganglion cell axons at the optic disc, creating the optic nerve. The optic nerve, or cranial nerve II, exits the posterior end of the eyeball, and travels posteriorly along the ventral surface of the brain. Like all other cranial nerves, the optic nerve is paired, meaning there is one for each eye. Both optic nerves merge at a spot called the optic chiasm, then diverge yet again as they travel posteriorly towards the thalamus.
The axonal connections in the optic nerve are not quite as simple as its anatomical appearance. From each optic nerve, some of the nerve fibers cross the midline (decussate), headed towards the contralateral hemisphere. Other nerve fibers meet at the optic chiasm, but then project into the ipsilateral hemisphere.
Prior to entering the brain, axons from the nasal portion of each retina cross the midline at the optic chiasm but the temporal portion of each retina does not cross at the optic chiasm.
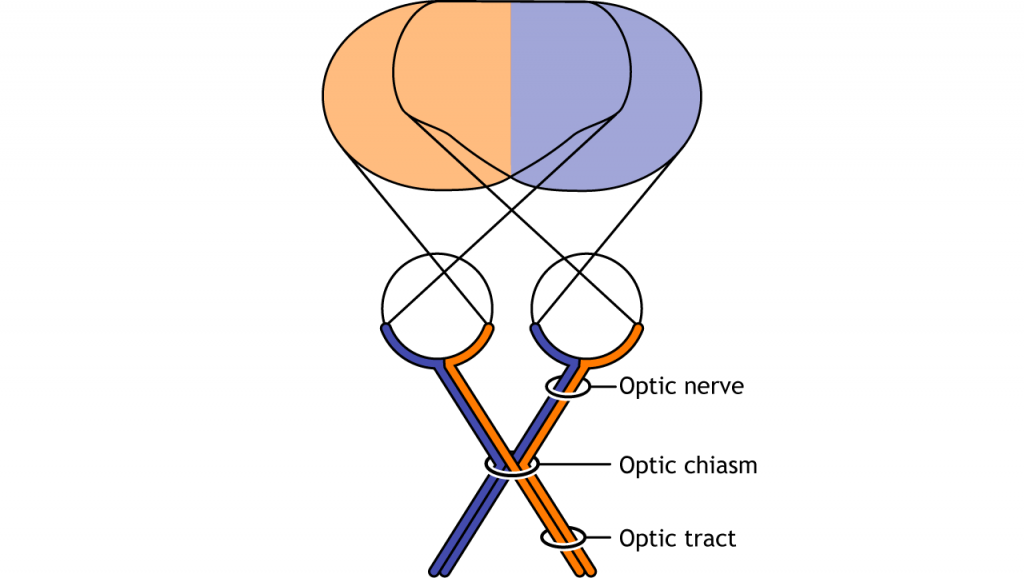
View the optic nerve (cranial nerve II) using the BrainFacts.org 3D Brain
Since the axons from the nasal retina cross to the opposite side of the nervous system but the temporal retina axons do not, this leads to the brain processing input from the contralateral (opposite side) visual hemifield. Therefore, the right side of the brain receives visual information from the left hemifield and vice versa. The easy way to keep track of this unusual system is to remember that all information from the left visual field enters the right hemisphere of the brain, while visual information from the right visual field enters the left hemisphere of the brain.
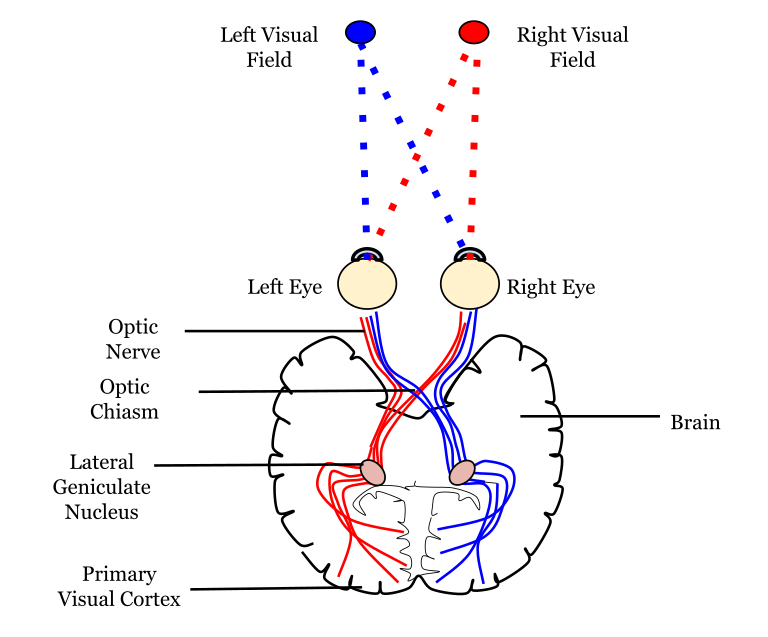
Pathways from the Retina
The retinal ganglion cells that exit the retina and project into the brain can take different paths. The retinofugal projection (fugal means “to flee”) is one of the major projection paths of the retinal ganglion cells. Most retinal output projects to the lateral geniculate nucleus of the thalamus and then to the primary visual cortex, however there are subsets of cells that are routed through other non-thalamic pathways.
Non-Thalamic Pathways
Not all of the axons convey direct visual information into the thalamus for visual perception. Some ganglion cells project to the superior colliculus, a midbrain region via the retinotectal pathway (recall that the superior colliculus is a structure of the tectum). This pathway communicates with motor nuclei and is responsible for pupillary control. Specifically, it is responsible for movements that will orient the head and eyes toward an object to focus the object in the center of the visual field, the region of highest visual acuity.
A subset of specialized retinal ganglion cells project to the suprachiasmatic nucleus in the hypothalamus through the retinohypothalamic tract (starts in the retina, ends in the hypothalamus). It does not carry any conscious visual information. The retinohypothalamic tract conducts light information from a small group of intrinsically-photosensitive retinal ganglion cells. This structure functions to help the body adapt its sleep-wake cycle in the face of changing day-night patterns This region is critical for circadian rhythms and the sleep/wake cycle.
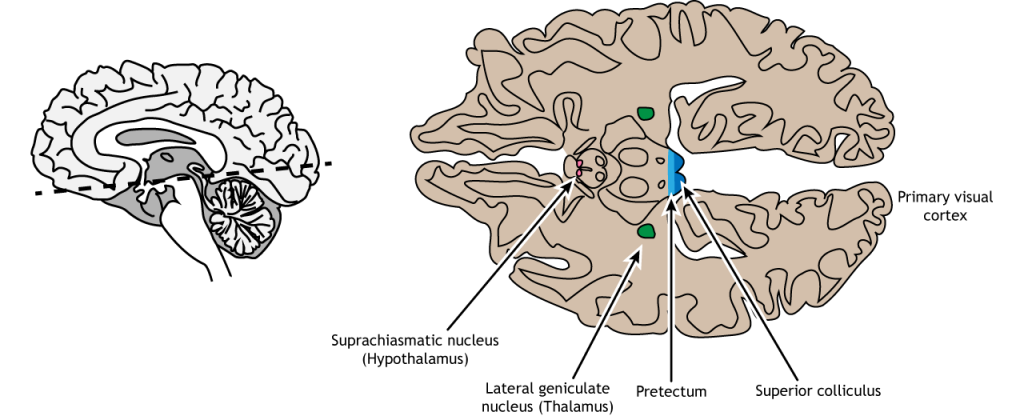
View the hypothalamus using the BrainFacts.org 3D Brain
View the midbrain using the BrainFacts.org 3D Brain
Thalamic Pathway: Lateral Geniculate Nucleus
In conscious visual perception, the first synapse of the optic nerve is formed in the thalamus at a subregion called the lateral geniculate nucleus, or LGN. The optic tract enters the brain and ascends to synapse in the lateral geniculate nucleus of the thalamus. From there, axons project to the primary visual cortex, also called the striate cortex or V1, located in the occipital lobe.
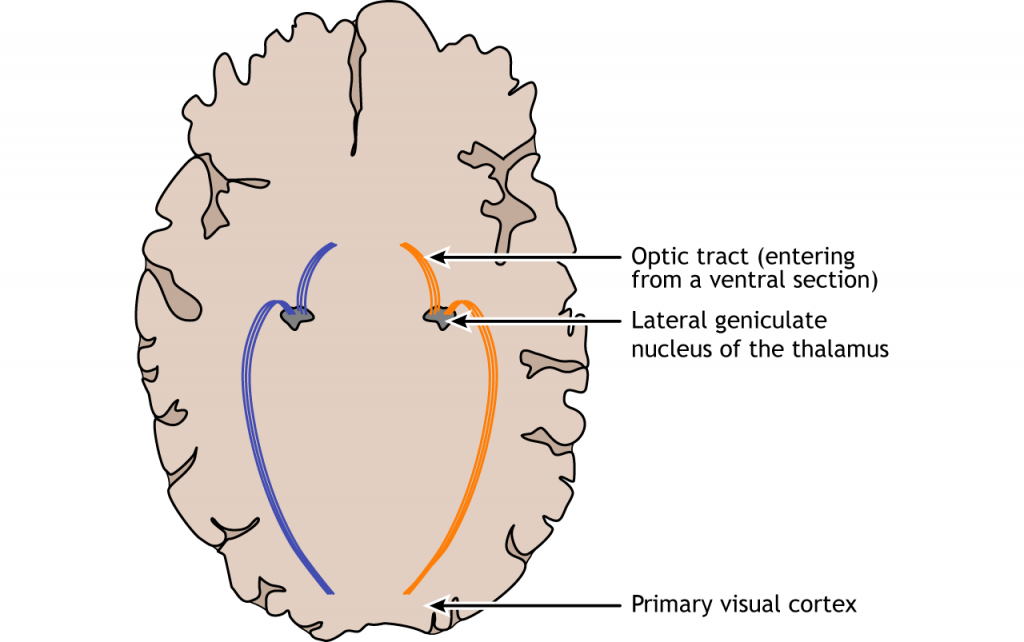
Lateral Geniculate Nucleus
The lateral geniculate nucleus of the thalamus (LGN) has six distinct layers when examined in cross section. The LGN serves as the first synaptic site of the retinal ganglion cells that exited the retina.
Importantly, visual information is separated at the level of the LGN such that visual information from each visual field is all processed in the contralateral LGN. This means that information from the left visual hemifield, collected via the right temporal retina and the left nasal retina will all be processed within the right LGN. Information from the right visual hemifield, collected via the left temporal retina and the right nasal retina will all be processed by the left LGN.
Within the LGN, input coming from each eye is kept separate by the layers of the LGN. For example, notice that half of the layers of the right LGN process information from the right eye and the other half of the layers process information from the left eye.
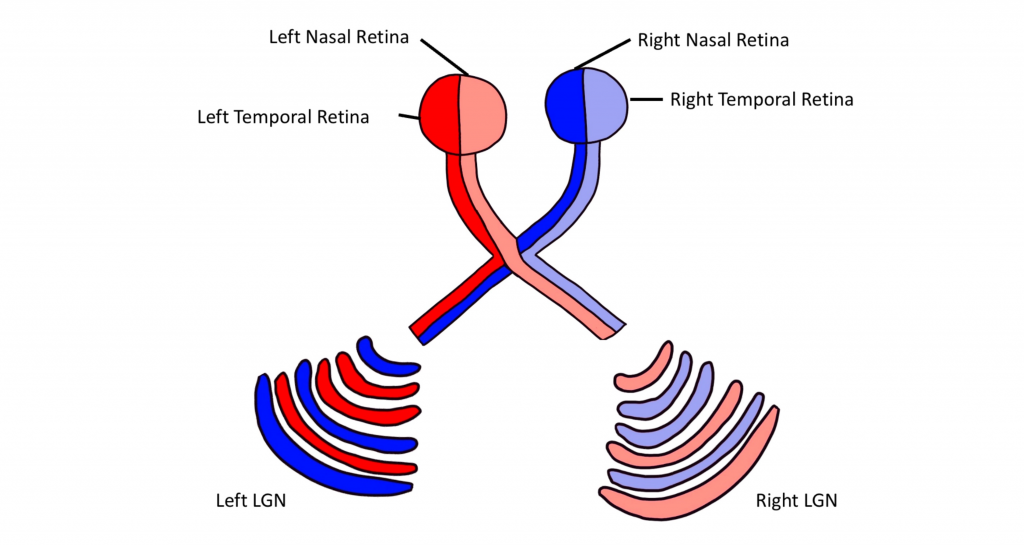
The outputs of the LGN are a series of axonal bundles called the optic radiations. From the LGN of the thalamus, the optic radiations project to the occipital lobe at the caudal (posterior) end of the brain. Once visual information travels into the cortex, the process is less about sensation and mostly about perception.
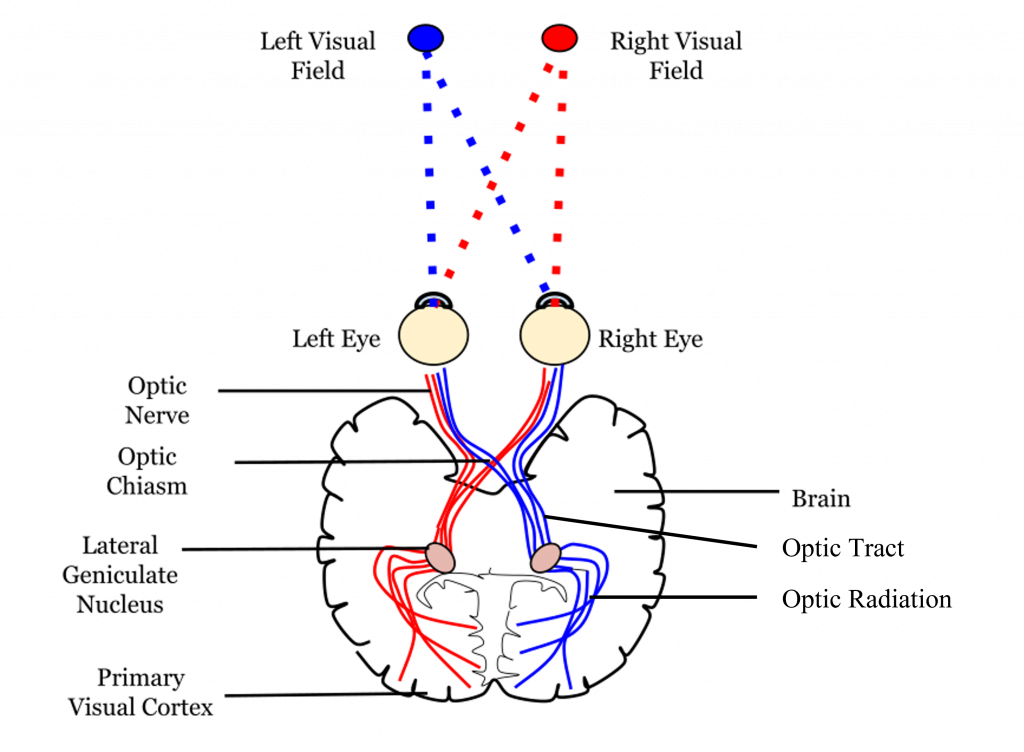
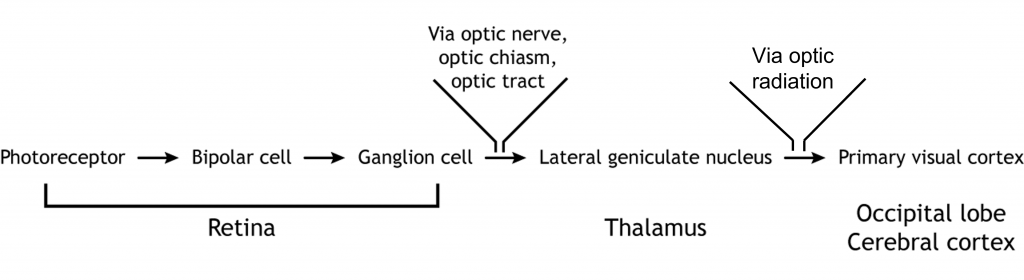
View the thalamus using the BrainFacts.org 3D Brain
Striate Cortex (Primary Visual Cortex)
The outputs of the LGN are axons which form synapses in the primary visual cortex, which is also called V1 or the striate cortex. ‘Striate’ means ‘stripe’ and is named due to the presence of a large white stripe that can be seen in unstained tissue during surgical dissection. This white stripe is the bundle of incoming optic tract axons, which are heavily myelinated.
Each neuron in V1 receives visual information from a specific patch of retinal cells. This organizational pattern, where a section of retinal inputs map onto neurons of a specific section of V1, is called retinotopic organization. This retinotopic organization is conserved from the retina to the LGN, and finally to the primary visual cortex. Visual information from the fovea, despite being only 1% of the total visual field, takes up about half of all neurons in V1. After processing in V1, visual information is passed along to other cortical areas that contribute to various aspects of visual perception.
View the primary visual cortex using the BrainFacts.org 3D Brain
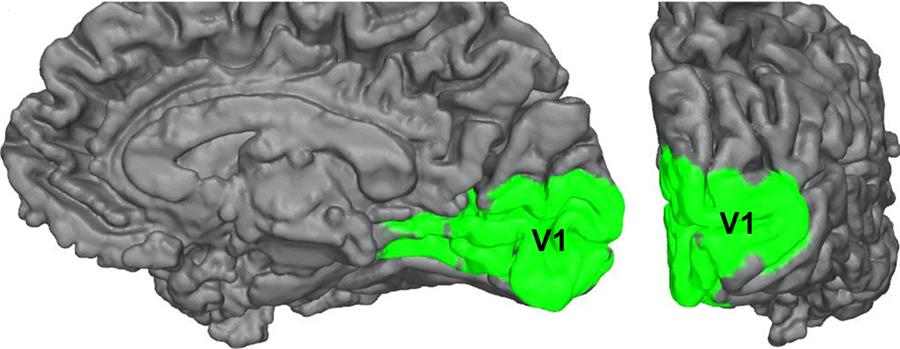
Cortical Layers
The cortex of the brain is arranged into six layers, named with the Roman numerals I-VI. The cells within these layers have different morphology. These cortical layers are especially visible within the striate cortex (V1). Within this region, the thickness of the cortex between the pia mater that is in contact with the top of the cortex and the white matter underlying the cortex is very thin (around 2 mm in thickness).
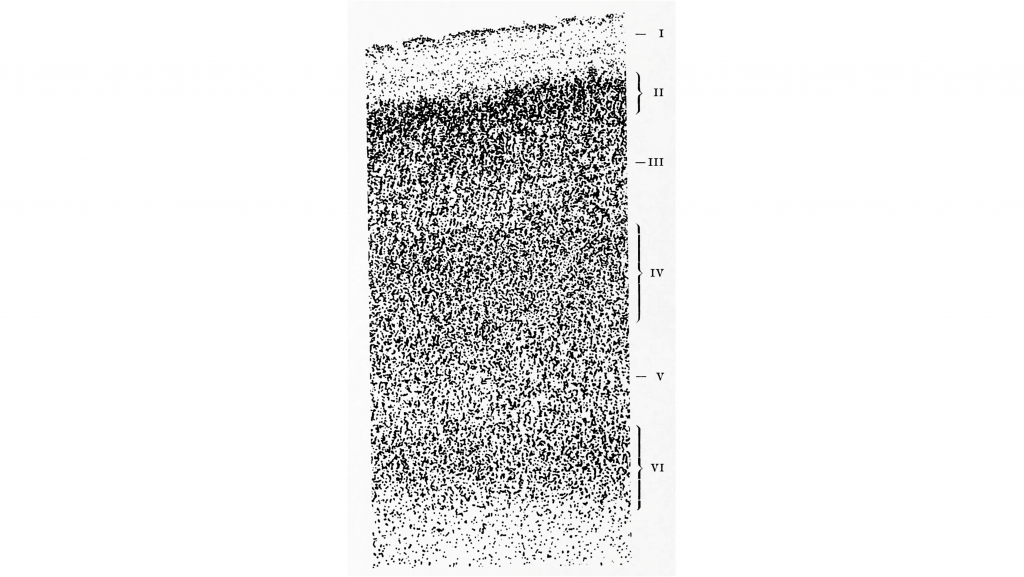
These cells within the six layers of the cortex are arranged in what are called ‘cortical columns’. This arrangement was discovered by Dr. Vernon Mountcastle in the 1950s. You can imagine that due to the directionality of communication in neurons, that information travels up and down the cells within a single cortical column.
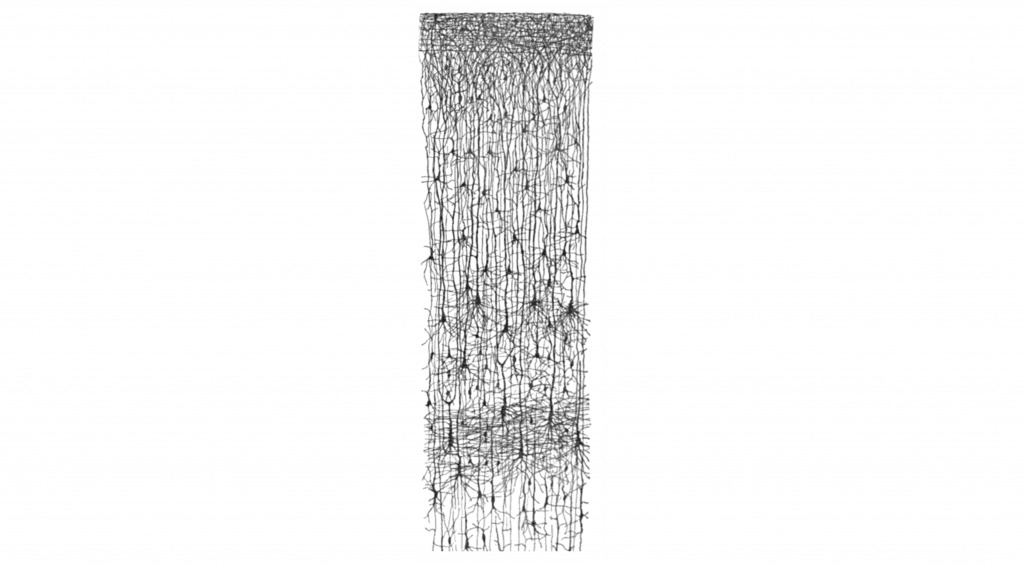
Features of the Primary Visual Cortex: Contributions of David Hubel and Torsten Wiesel
Dr. David Hubel and Dr. Torsten Wiesel followed up on this critical discovery by Mountcastle to describe neuronal processing within the visual cortex. Their discoveries in this field led to them being awarded the Nobel Prize in the field of Physiology or Medicine in 1981. Highlighted below are some of their major contributions.
Orientation Selectivity
Hubel and Wiesel used microelectrodes to map the functioning of the visual cortex. In one of their famous experiments, Hubel and Wiesel used anesthetized cats to determine how the visual cortex processes visual information. During their experiment, they were showing the anesthetized cat different visual stimuli through the projection of slides with different images onto a screen while recording from neurons within the visual cortex. Due to when this experiment was done, these slides were large and plastic and had to be physically inserted into a projector. In the process of inserting these slides into the projector, a bar of light was projected onto the screen by the edge of the slide. Quite by surprise, the act of changing the slides (and the bar of light that resulted) caused the neurons within the cat brain to fire action potentials. Hubel and Wiesel determined that the stimulus causing the neurons to fire was the angle of the bar of light projected on the screen.
In fact, the neurons within the visual cortex responded best to a line in a specific orientation and the firing rate of the neuron increased as the line rotated toward the “preferred” orientation. The firing rate is highest when the line is in the exact preferred orientation and different orientations are preferred by different neurons. This discovery was due to some great luck for Hubel and Wiesel as they were recording from neurons that had a preferred orientation that exactly matched the angle of changing the slides in their projector!
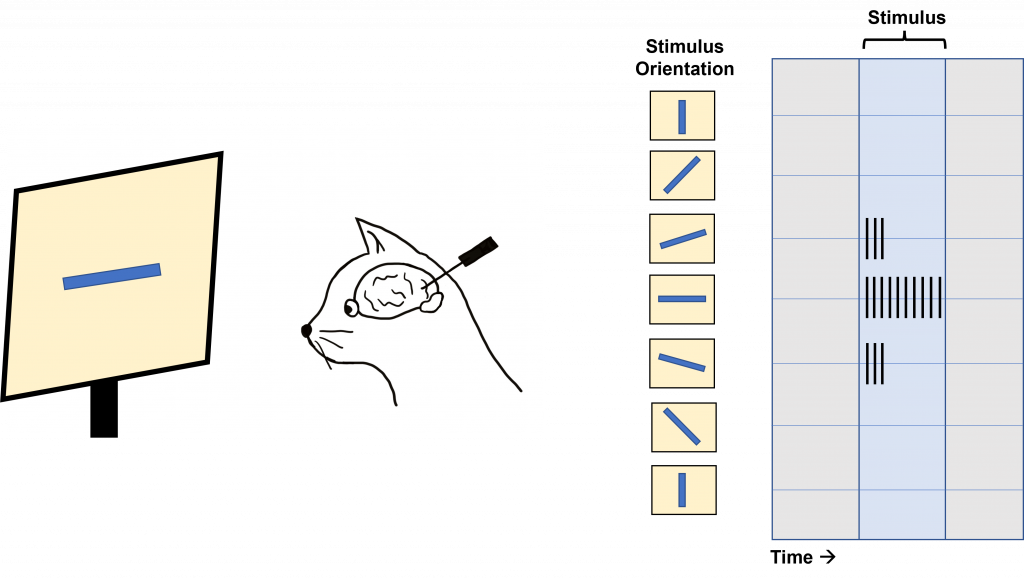
This orientation selectivity within the visual cortex is the same for all neurons located within a single cortical column. This means that the cells in each cortical column will respond optimally to a line at a preferred orientation. So, if a recording electrode is inserted vertically through the cortex to record from cells within a single cortical column, all cells will respond optimally to the same sensory stimulus. If the recording electrode is inserted horizontally, such that it records across multiple cortical columns, each cortical column will respond optimally to a different sensory stimulus.
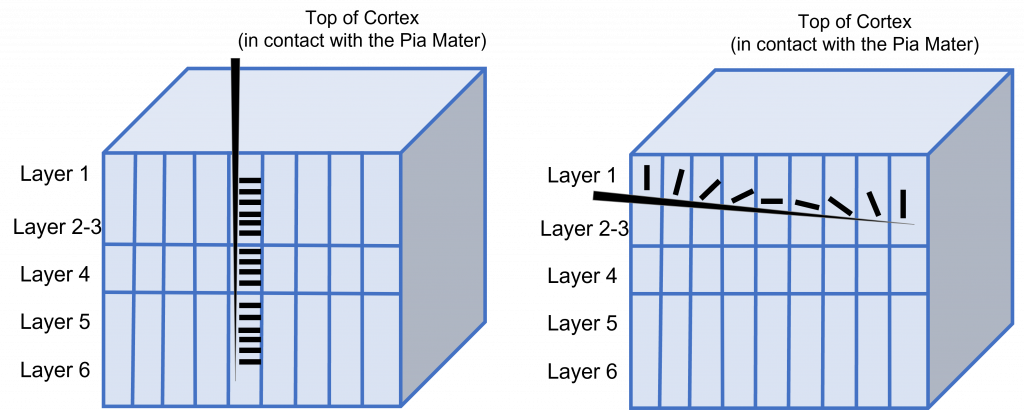
Ocular Dominance Columns
Another experiment by Hubel and Wiesel determined the existence of ocular dominance columns, or columns of neurons within the visual cortex that respond preferentially to either the left or right eye.
For this experiment, Hubel and Wiesel injected a radioactive amino acid into one of the eyes of a monkey. This amino acid then travels by anterograde transport through the retinal ganglion cells of the eye through the first synapse at the lateral geniculate nucleus of the thalamus, and then through the next synapse with the striate cortex. Radioactivity within the striate cortex can then be visualized through the process of autoradiography.
In an autoradiograph image of the striate cortex, there are alternating areas that appear white in color with areas that appear black in color (similar to the stripes on a zebra). The areas of the cortex that appear white are the areas that took up the radioactive amino acid and are processing information from the injected eye. The areas of the cortex that appear black are areas that do not have the radioactive amino acid and are processing information from the eye that was not injected. Recall, that although information from the left visual hemifield is processed on the right side of the brain, the left visual hemifield information is collected from both eyes via the left nasal retina and right temporal retina. Therefore, both eyes are processed across both the left and right visual cortex.
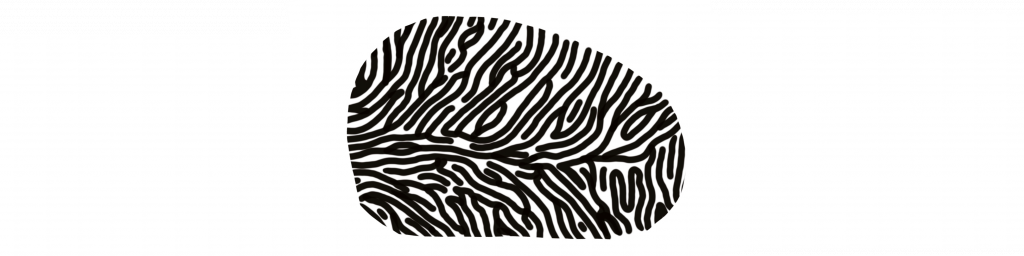
Interestingly, in newborn cats that had not had any visual experience, they did not observe the ocular dominance columns. Instead, they saw that the inputs of the left and right eye overlapped substantially within the visual cortex. Hubel and Wiesel were interested in understanding how these ocular dominance columns develop between infancy and adulthood and whether their development was dependent on visual experience.
For their experiment, they took a kitten immediately after birth, and sewed the right eye of the kitten closed, so that the eye could no longer have any visual experience, a condition called monocular deprivation (‘mono’ meaning ‘one’ and ‘ocular’ meaning ‘eye’). After this six-week period of monocular deprivation, the eye of the cat was reopened so that it could take in the visual environment. A control group of cats had normal visual experience for this same six-week period.
In control cats that did not experience monocular deprivation, Hubel and Wiesel found that they developed normal ocular dominance columns at six weeks post birth. The cats that experienced monocular deprivation for the first six weeks of life, however, showed abnormal development of the ocular dominance columns. The columns that normally served the right eye (that was sewn shut) shrank in size, and the neighboring columns that served the left eye grew into the unoccupied cortical space. Hubel and Wiesel were able to conclude that visual experience was necessary for the ocular dominance columns to develop. In fact, they found that there was a critical period in cat visual development in the first six weeks of life where visual experience is required for normal visual development.
Importantly, these structural changes also translated into functional changes for the vision of the cat. Cats that experienced monocular deprivation had decreased visual function in the eye that was sewn shut for the rest of the cat’s life. Even though the sewn shut eye was reopened after the critical period, the development of the ocular dominance columns in the brain was already complete and thus the brain could never process visual information from the sewn shut eye.
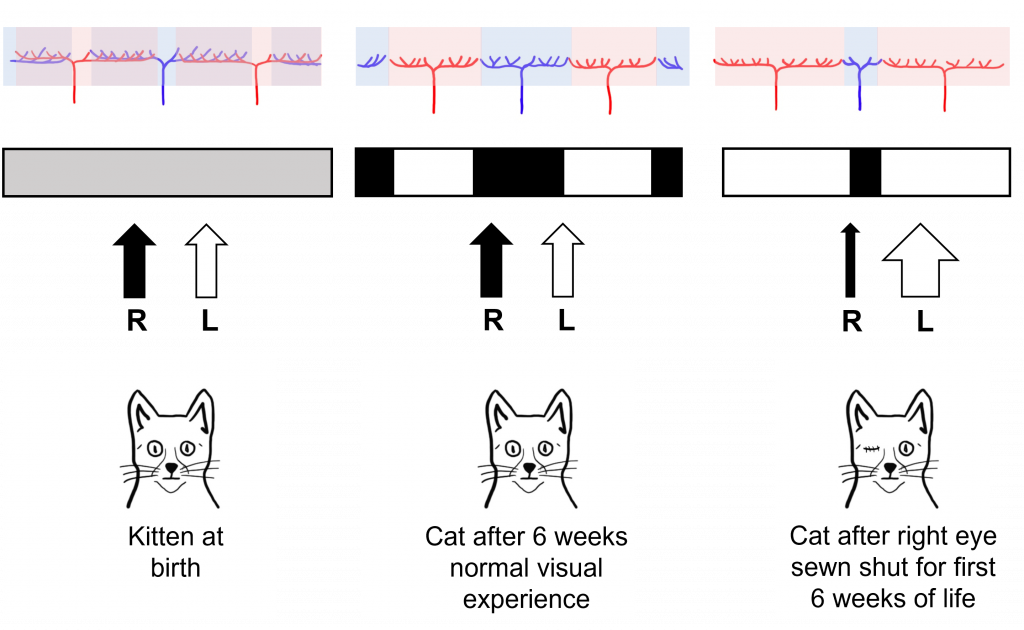
Higher-Level Processing of Sensory Information
Sensory system processing of input does not end upon reaching the primary sensory cortex in any sensory system. Information typically gets sent from the primary sensory cortex to other sensory association regions throughout the brain. The characteristics of sensory information becomes more complex as this higher-level processing occurs.
Post-Striatal Processing
In the visual system, there are two broad streams of information that leave the striate cortex. Visual information passes through two streams of communication: the dorsal stream and the ventral stream. In the ventral stream, information travels from the primary visual cortex down through the inferior temporal lobe is responsible for determining object recognition, or what an object is. Differentiating between an apple and a person occurs in this stream. In the dorsal stream, information travels from the striate cortex up through the parietal lobe and is responsible for motion or spatial components of vision.
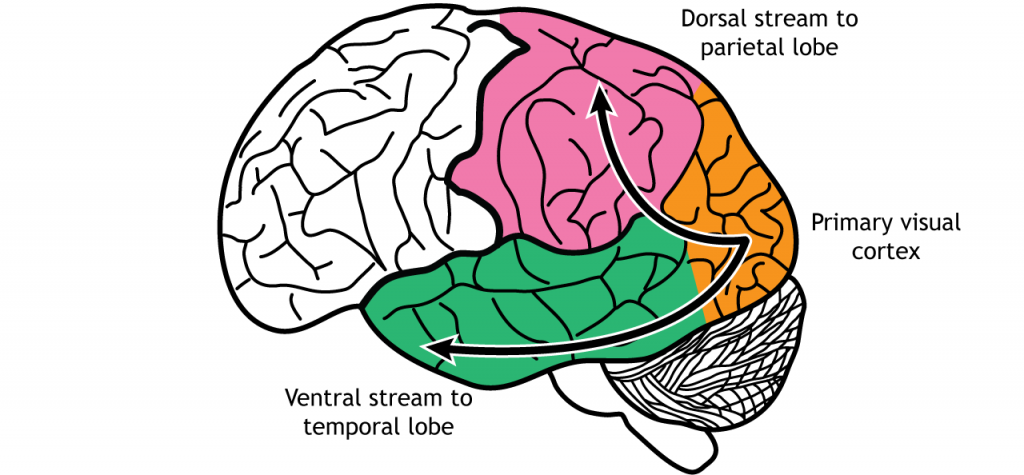
Dorsal Stream
The dorsal stream is described as the “where” pathway because these structures help us identify where objects are located in the space around us. One of the most important regions in the dorsal pathway is region MT, also called V5, which contributes to perception of motion. In this region, neurons are preferentially activated by a specific direction of movement by an object – for example, left to right or up to down. As an example, remember the receptive fields in the primary visual cortex were activated by lines at a specific orientation. Like that, in V5, the neurons would be activated by lines moving in a specific direction.
As information continues to be processed through the dorsal stream, the neurons become selective for more complex motions. The dorsal stream is also important for processing our actions in response to visual stimulation, for example, reaching for an object in the visual field or navigating around objects while walking.
These structures also guide us when we move through our environments, contributing to our sense of spatial awareness. For example, a task such as reaching out to grab an object in front of you uses a combination of these features, so this task is guided largely by dorsal stream structures.
Figure 30.20. Area MT, also called V5, is an early processing region of the dorsal stream through the parietal lobe. Neurons in the region are activated by direction of an object in a specific direction. ‘Area MT’ by Casey Henley is licensed under a Creative Commons Attribution Non-Commercial Share-Alike (CC BY-NC-SA) 4.0 International License.
Ventral Stream
The ventral stream is the “what” pathway, and helps in the identification of objects that we see. Object identification is a key function of our visual system. The ventral visual stream is responsible for this process. Further, ventral stream structures are important for visual memory. Like the more complex activation characteristics of region MT in the dorsal stream, neurons in Area V4 in the ventral stream show more complex receptive fields and show sensitivity to shape and color identification. In fact, there is a rare clinical condition in humans called achromatopsia, in which individuals have partial or complete loss of color vision. These individuals still have normal functioning cones within the retina, and normal LGN and primary visual cortex function. Instead, they typically have damage to occipital and temporal lobes, where the ventral stream is located.
A major output of area V4 is area IT, located within the inferior temporal lobe. As information travels to area IT it continues to be processed and differentiation of objects occurs. Neurons located in area IT are important in learning and memory in visual perception (have I seen that before?), and have been found to respond to colors and shapes.
Another region within the ventral stream called the fusiform face area, located in the fusiform gyrus, which lies on the ventral aspect of the temporal lobe, contains neurons that are activated by faces and can be specialized to one specific face. When the visual system senses these complex stimuli, those signals get processed through these ventral stream pathways. These incoming stimuli are compared with the memories stored in the ventral stream, and this comparison contributes to our capacity for perception and identification.
The two streams are not independent of each other. Rather, successful organisms require the melding of both components of visual perception. For example, imagine you are a prehistoric organism living in a food-scarce environment. Approaching a small berry tree, you would use ventral stream structures to correlate the berries with memories: Did these berries taste good and give me the calories I need to survive? Or did these berries make me violently ill, and are therefore probably poisonous? If they are the delicious berries that I want, I will use the dorsal stream structures to take note of their precise location so I can reach out for them and pick the berries . In this example, proper interaction of the dual streams contributes to goal-driven actions.
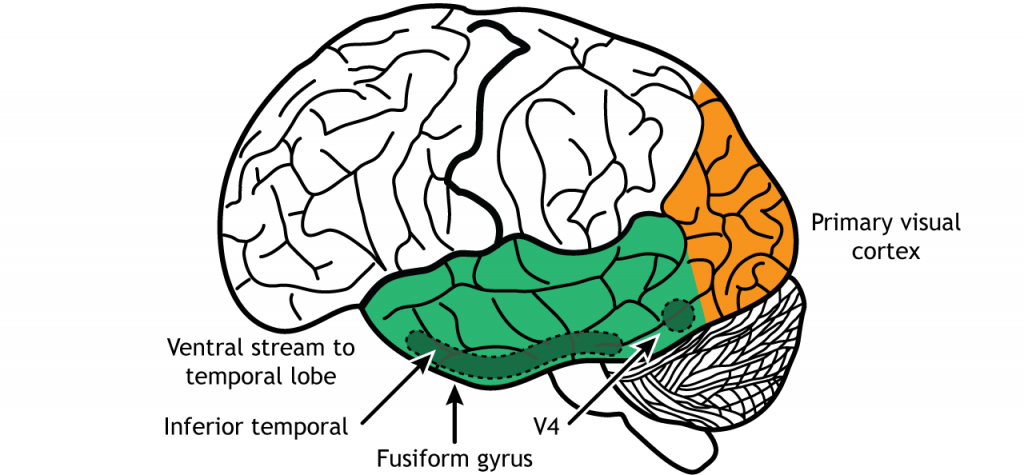
The inferior temporal lobe also makes reciprocal connections with the structures in the limbic system. The limbic system plays an important role in processing emotions and memory, both of which are significant components to visual perception. The amygdala ties visual stimuli with emotions and provides value to objects. A family member will have emotional ties that a stranger will not. The hippocampus is responsible for learning and memory and helps establish memories of visual stimuli.
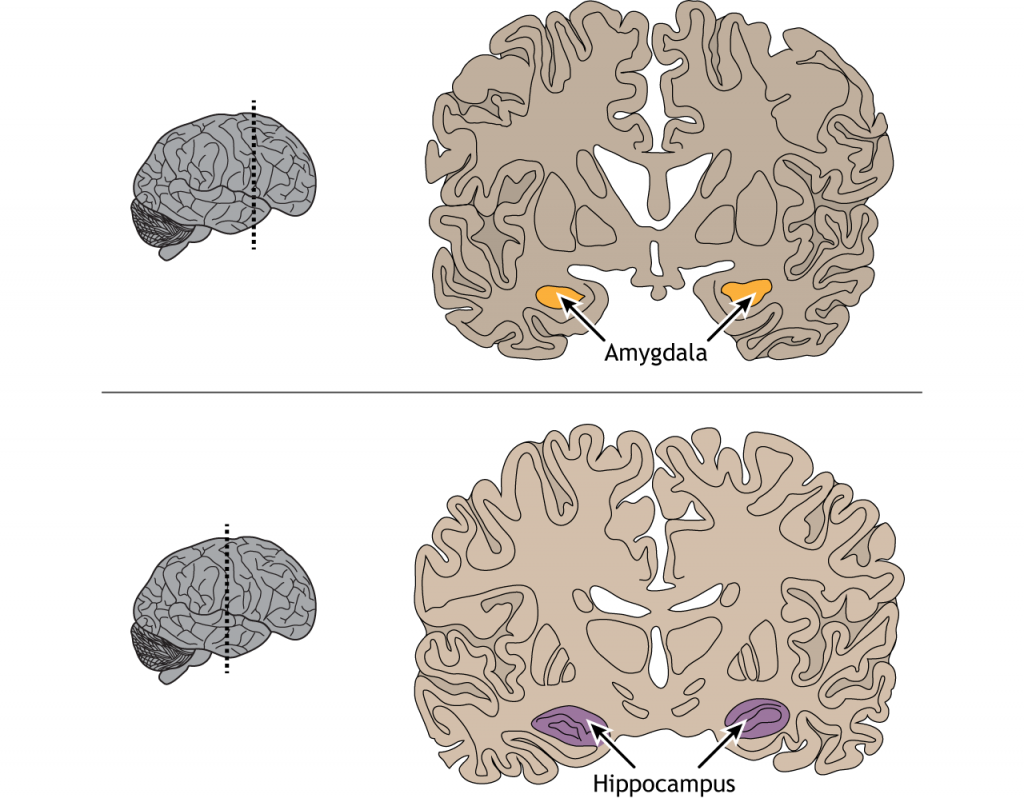
View the amygdala using the BrainFacts.org 3D Brain
View the hippocampus using the BrainFacts.org 3D Brain
Key Takeaways
- The nasal and temporal retinal regions are responsible for viewing specific regions of the visual field
- Some retinal projections cross the midline at the optic chiasm, causing the left side of the brain to process the right visual hemifield and vice versa
- The retinal axons synapse in the lateral geniculate nucleus of the thalamus. Information then travels to the primary visual cortex
- Receptive fields and the preferred visual stimuli for neuron activation become more complex as information moves through the visual pathway
- Primary visual cortex neurons have linear receptive fields are are activated by a line in a specific orientation
- Area MT / V5 is activated by motion in a specific direction
- Area V4 is activated by specific shapes and colors
- The fusiform gyrus is activated by faces
- The retina also projects to midbrain regions
- Ocular dominance columns that process information from either the left or right eye develop during a critical period of development
Test Yourself!
Attributions
Portions of this chapter were remixed and revised from the following sources:
- Foundations of Neuroscience by Casey Henley. The original work is licensed under a Creative Commons Attribution-NonCommercial-ShareAlike 4.0 International License
- Open Neuroscience Initiative by Austin Lim. The original work is licensed under a Creative Commons Attribution-NonCommercial 4.0 International License.
Media Attributions
- neural pathway from the retina © Mads00 is licensed under a CC BY-NC-SA (Attribution NonCommercial ShareAlike) license
- Lateral Geniculate Nucleus © Valerie Hedge is licensed under a CC BY-NC-SA (Attribution NonCommercial ShareAlike) license
- optic radiation © Mads00 adapted by Valerie Hedges is licensed under a CC BY-NC-SA (Attribution NonCommercial ShareAlike) license
- Visual pathway © Casey Henley adapted by Valerie Hedges is licensed under a CC BY-NC-SA (Attribution NonCommercial ShareAlike) license
- Human Primary Visual Cortex © Geyer S, Weiss M, Reimann K, Lohmann G and Turner R is licensed under a CC BY (Attribution) license
- Laminar organization of the cortex © Korbinian Brodmann adapted by Valerie Hedges is licensed under a Public Domain license
- Cajal Cortex Drawings © Santiago Ramon y Cajal adapted by Valerie Hedges is licensed under a Public Domain license
- Orientation selectivity © Valerie Hedges is licensed under a CC BY-NC-SA (Attribution NonCommercial ShareAlike) license
- Orientation selectivity in cortical columns © Valerie Hedges is licensed under a CC BY-NC-SA (Attribution NonCommercial ShareAlike) license
- Ocular dominance columns © Valerie Hedges is licensed under a CC BY-NC-SA (Attribution NonCommercial ShareAlike) license
- Development of ocular dominance columns © Valerie Hedges is licensed under a CC BY-NC-SA (Attribution NonCommercial ShareAlike) license
everything we can see without moving our head or eyes
Structure in the middle of the retina
the area of each retina that is closest to the nose
the areas of the retina that are closes to the temples on the side of the head
visual space that can only be viewed by one eye
visual space that can be viewed by both eyes
Behind; toward the back
Toward the bottom of the brain or the front of the spinal cord
Where the optic nerves merge
denotes the side of the body opposite to the side where a structure started
occurs on the same side of the body
Toward the back of the brain or the bottom of the spinal cord
columns of neurons that respond preferentially to either the left or right eye
Movement from the cell body to the axon terminals
a window of time where a system has heightened sensitivity to particular stimuli in order to develop properly