8 Electrical Activity in Neurons
The membrane potential will always fall into one of the following categories:
- Resting membrane potential
- Graded Potentials (postsynaptic potentials)
- Action potentials
When the neuron is at rest, there is a baseline level of ion flow through leak channels. However, the ability of neurons to function properly and communicate with other neurons through action potentials relies on ion flow through channels other than the non-gated leak channels. We will cover how these channels open in a later lesson. This chapter will examine ion flow through these channels after a stimulus and how the membrane potential changes in response.
Postsynaptic Potentials (Graded Potentials)
Postsynaptic potentials (also called graded potentials) are changes in membrane potential that move the cell away from its resting state. For our purposes, postsynaptic potentials are measured in the dendrites and cell bodies. Ion channels that are opened by a stimulus allow brief ion flow across the membrane.
A stimulus can range from neurotransmitters released by a presynaptic neuron, changes in the extracellular environment like exposure to heat or cold, interactions with sensory stimuli like light or odors, or other chemical or mechanical events. The change in membrane potential in response to the stimulus will depend on which ion channels are opened by the stimulus.
Depolarization: Excitatory Postsynaptic Potentials (EPSPs)
An Excitatory Postsynaptic Potential (EPSP) occurs when sodium channels open in response to a stimulus. The electrochemical gradient drives sodium to rush into the cell. When sodium brings its positive charge into the cell, the cell’s membrane potential becomes more positive, or depolarizes. This change is called a depolarization because the cell’s membrane potential is moving toward 0 mV, and the membrane is becoming less polarized. At 0 mV, there is no potential or polarization across the membrane, so moving toward 0 would be a decrease in potential. This depolarization increases the likelihood a neuron will be able to fire an action potential, which makes this ion flow excitatory. Therefore, an EPSP is an excitatory change in the membrane potential of a postsynaptic neuron.
A postsynaptic potential is typically brief, with ion channels closing quickly after the stimulus occurs. If there is not another stimulus, the cell will return to the resting membrane potential.
Animation 8.2. When a stimulus opens sodium channels, sodium rushes into the cell because the equilibrium potential of sodium is +60 mV. This causes an excitatory depolarization called an excitatory postsynaptic potential (EPSP). After the stimulus, the ion channels close, and the membrane potential returns to rest. The dotted, blue channels represent sodium channels; the striped, green channels represent potassium channels; the solid yellow channels represent chloride channels. ‘EPSP’ by Casey Henley is licensed under a Creative Commons Attribution Non-Commercial Share-Alike (CC BY-NC-SA) 4.0 International License. View static image of animation.
Hyperpolarization: Inhibitory Postsynaptic Potentials (IPSPs)
An inhibitory postsynaptic potential, or IPSP, on the other hand, is caused by the opening of chloride channels. The equilibrium potential of chloride is -65 mV, so if the neuron is at rest at -60 mV, the electrochemical gradients drive chloride to flow into the cell when chloride channels open. Chloride brings its negative charge into the cell, causing the cell’s membrane potential to become more negative, or hyperpolarize. This change is called a hyperpolarization because the cell’s membrane potential is moving away from 0 mV, and the membrane is becoming more polarized. An IPSP decreases the likelihood a neuron will be able to fire an action potential, which make this ion flow inhibitory. Therefore, an IPSP is an inhibitory change in the membrane potential of a postsynaptic neuron.
An IPSP can also be caused by the opening of potassium channels. The equilibrium potential of potassium is -80 mV, so if the neuron is at rest at -60mV, the electrochemical gradients drive potassium out of the cell when potassium channels open. As positively charged potassium ions leave the cell, this causes the cell’s membrane potential to hyperpolarize. This demonstrates the importance of the equilibrium potential in driving ion movement.
Like an EPSP, an IPSP is also typically brief, and the membrane potential will return to rest if no additional stimulation occurs.
Animation 8.3. When a stimulus opens chloride channels, and the resting membrane potential is more positive than chloride’s equilibrium potential of -65 mV, chloride rushes into the cell. This causes an inhibitory hyperpolarization called an inhibitory postsynaptic potential (IPSP). After the stimulus, the ion channels close, and the membrane potential returns to rest. The dotted, blue channels represent sodium channels; the striped, green channels represent potassium channels; the solid yellow channels represent chloride channels. ‘IPSP’ by Casey Henley is licensed under a Creative Commons Attribution Non-Commercial Share-Alike (CC BY-NC-SA) 4.0 International License. View static image of animation.
The Resting Membrane Potential is Critical
In the previous example, the resting membrane potential of that cell was -60 mV, so chloride moved into the cell. If the resting membrane potential was instead equal to chloride’s equilibrium potential of -65 mV, then chloride would be at equilibrium and move into and out of the cell; there would be no net movement of the ion. Even though this would lead to no change in membrane potential, the opening of chloride channels continues to be inhibitory. Increased chloride conductance would make it more difficult for the cell to depolarize and to fire an action potential.
Animation 8.4. If the cell is at rest at chloride’s equilibrium potential, when a stimulus opens the chloride channels, there will be no net movement of chloride in either direction because chloride will be at equilibrium. Since there is no net movement, there will also be no change in membrane potential because there is an equal amount of ion flow into and out of the cell. The dotted, blue channels represent sodium channels; the striped, green channels represent potassium channels; the solid yellow channels represent chloride channels. ‘IPSP at Equilibrium’ by Casey Henley is licensed under a Creative Commons Attribution Non-Commercial Share-Alike (CC BY-NC-SA) 4.0 International License. View static image of animation.
If the resting membrane potential of the cell was more negative than chloride’s equilibrium potential—for example, at -70 mV—then chloride would leave the cell in order to move the membrane potential toward -65 mV. This would result in a depolarization of the membrane potential. However, the overall effect is still inhibitory because once the cell reaches -65 mV, the driving forces acting on chloride would try to keep the cell at that membrane potential, making it more difficult for the cell to depolarize further and fire an action potential.
A good rule of thumb is to remember that opening of sodium channels is excitatory whereas opening of potassium or chloride channels is typically inhibitory.
Animation 8.5. If the cell is at rest at chloride’s equilibrium potential, chloride will leave the cell when a stimulus opens the chloride channels, removing its negative charge. This causes a depolarization in the membrane potential, but it is still inhibitory since chloride movement will try to keep the cell near -65 mV. The dotted, blue channels represent sodium channels; the striped, green channels represent potassium channels; the solid yellow channels represent chloride channels.‘Inhibitory Depolarization’ by Casey Henley is licensed under a Creative Commons Attribution Non-Commercial Share-Alike (CC BY-NC-SA) 4.0 International License. View static image of animation.
Summation of Inputs
If an excitatory stimulus is followed by additional excitatory stimuli, the sodium channels will either remain open or additional sodium channels will open. The increased sodium conductance will cause the EPSPs to summate, depolarizing the cell further than one EPSP alone. Each neuron has a threshold membrane potential at which the cell will fire an action potential. The summation of EPSPs causes the neuron to reach that threshold.
Animation 8.6. Excitatory stimuli that occur quickly in succession lead to summation of EPSPs. This leads to increased depolarization of the membrane potential compared to a single EPSP. The dotted, blue channels represent sodium channels; the striped, green channels represent potassium channels; the solid yellow channels represent chloride channels. ‘Summated EPSP Ion Flow’ by Casey Henley is licensed under a Creative Commons Attribution Non-Commercial Share-Alike (CC BY-NC-SA) 4.0 International License. View static image of animation.
Summation can occur in two ways. Temporal summation occurs when one presynaptic input stimulates a postsynaptic neuron multiple times in a row. Spatial summation occurs when multiple presynaptic inputs each stimulate the postsynaptic neuron at the same time. Both types of summation result in a depolarization of a higher magnitude than when only a single excitatory input occurs.
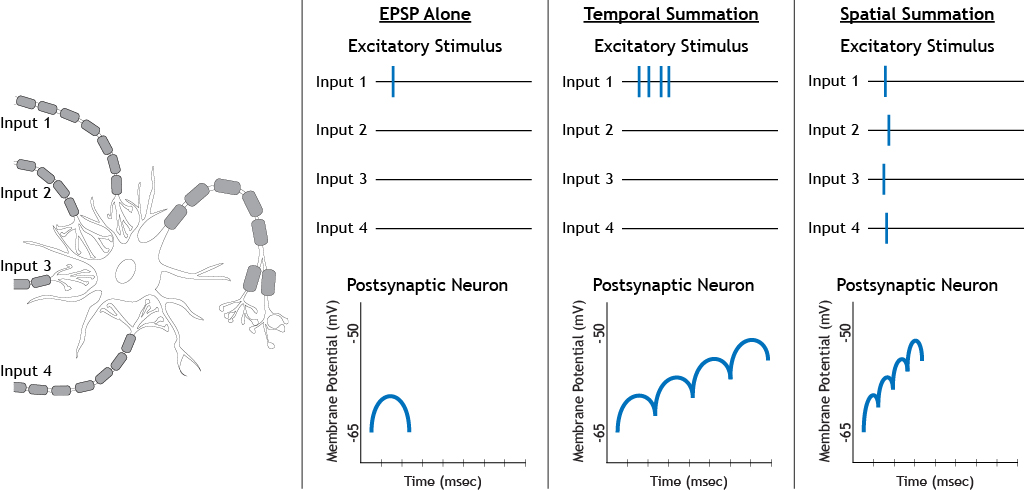
In addition to the summation of excitatory inputs, EPSPs can also summate with inhibitory inputs. The addition of an inhibitory stimulus will result in either a weaker depolarization compared to a single excitatory stimulus, or possibly no depolarization at all depending on the strength of the inhibitory input.
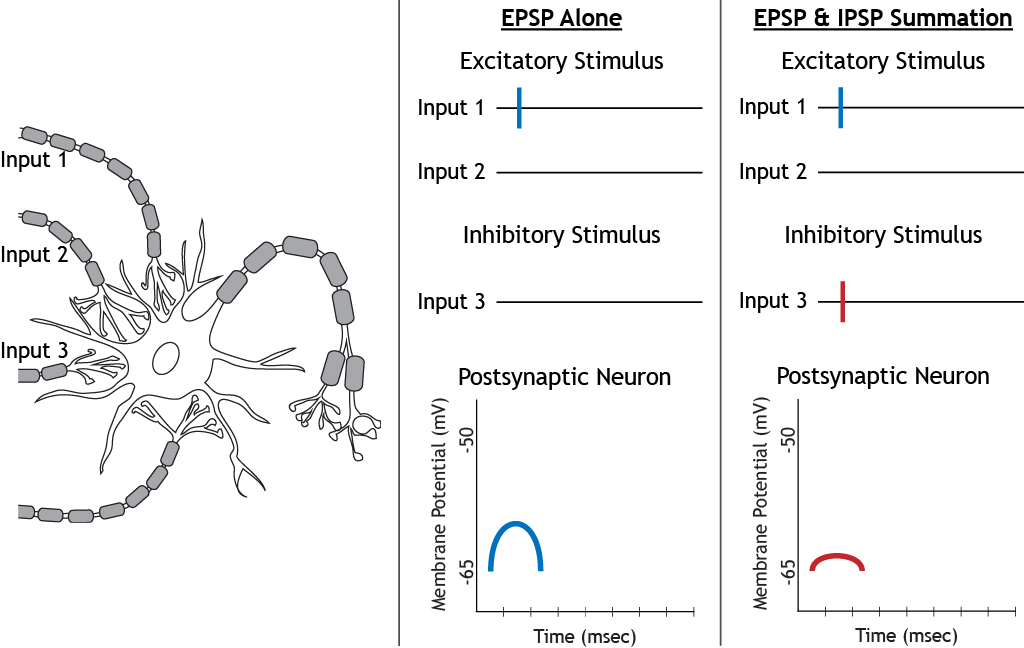
In the case of combined inhibitory and excitatory stimuli, both chloride and sodium channels will open. As sodium enters the cell, trying to move the membrane potential to +60 mV (the equilibrium potential of sodium) chloride will also enter, trying to keep the cell near -65 mV (the equilibrium potential of chloride).
Animation 8.7. When an inhibitory input and an excitatory input stimulate a postsynaptic neuron at the same time, chloride and sodium channels open. Due to the equilibrium potentials of the two ions, both will flow into the cell. Sodium tries to depolarize the cell, whereas chloride tries to keep the cell near rest. The dotted, blue channels represent sodium channels; the striped, green channels represent potassium channels; the solid yellow channels represent chloride channels. ‘EPSP and IPSP Ion Flow’ by Casey Henley is licensed under a Creative Commons Attribution Non-Commercial Share-Alike (CC BY-NC-SA) 4.0 International License. View static image of animation.
Key Takeaways
- Postsynaptic potentials occur in the dendrites or cell body
- Excitatory postsynaptic potentials are caused by sodium channels opening
- Inhibitory postsynaptic potentials are caused by chloride channels opening
- Since the resting membrane of a typical neuron is usually very close to chloride’s equilibrium potential, knowing and comparing these two values is important for determining direction of ion flow when chloride channels open
- Input effects, whether excitatory or inhibitory, can summate and affect the postsynaptic neuron’s membrane potential
Test Yourself!
Attributions
Portions of this chapter were remixed and revised from the following sources:
- Foundations of Neuroscience by Casey Henley. The original work is licensed under a Creative Commons Attribution-NonCommercial-ShareAlike 4.0 International License
Ion channels that are always open
The electrical signal transmitted by the axon of a neuron.
small changes in membrane potential that move the cell away from its resting state
When the membrane potential gets closer to zero
When the membrane potential gets further away from zero
add together
a specific membrane potential value that is more positive than resting membrane potential. Threshold potential can differ between cells.
Summation of synaptic inputs over time
Summation over space that occur at the same time