2 Ion Movement
Ion flow across the neuronal membrane is essential for neural activity, influencing the cell at rest and during communication. This process is tightly regulated by ion channels and driven by electrochemical gradients.
Phospholipid Bilayer Prevents Ion Movement
The neuronal membrane is composed of lipid molecules that form two layers. The hydrophilic heads of the molecules align on the outside of the membrane, interacting with the intra- and extracellular solution of the cell, whereas the hydrophobic tails are arranged in the middle, forming a barrier to water and water-soluble molecules like ions. This barrier is critical to neuron function.
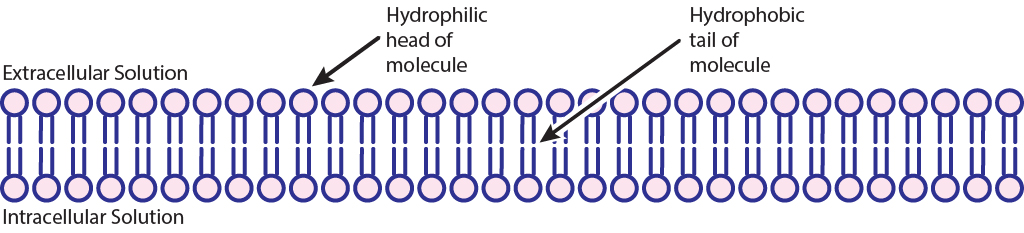
Ions move in predictable ways
Ion Channels Allow Ion Movement
Embedded throughout the neuronal membrane are ion channels. Ion channels are proteins that span the width of the cell membrane and allow charged ions to move across the membrane. Ions cannot pass through the phospholipid bilayer without a channel. Channels can be opened in a number of different ways. Channels that open and close spontaneously are called leak or non-gated channels. Channels that open in response to a change in membrane potential are called voltage-gated. Channels that open in response to a chemical binding are called ligand-gated. Other mechanisms like stretch of the membrane or cellular mechanisms can also lead to the opening of channels. Channels can be specific to one ion or allow the flow of multiple ions.

Ion channels control ion movement across the cell membrane because the phospholipid bilayer is impermeable to the charged atoms. When the channels are closed, no ions can move into or out of the cell. When ion channels open, however, then ions can move across the cell membrane.
Gradients Drive Ion Movement
Ions move in predictable ways. Concentration and electrical gradients drive ion movement. Ions will diffuse from regions of high concentration to regions of low concentration. Diffusion is a passive process, meaning it does not require energy. As long as a pathway exists (like through open ion channels), the ions will move down the concentration gradient.
In addition to concentration gradients, electrical gradients can also drive ion movement. Ions are attracted to and will move toward regions of opposite charge. Positive ions will move toward regions of negative charge, and vice versa.
For discussion of ion movement in this text, the combination of these two gradients will be referred to as the electrochemical gradient. Sometimes the concentration and electrical gradients driving ion movement can be in the same direction; sometimes the direction is opposite. The electrochemical gradient is the summation of the two individual gradients and provides a single direction for ion movement.
When Gradients Balance, Equilibrium Occurs
When the concentration and electrical gradients for a given ion balance, meaning they are equal in strength but in different directions, that ion will be at equilibrium. Ions still move across the membrane through open channels when at equilibrium, but there is no net movement in either direction meaning there is an equal number of ions moving into the cell as there are moving out of the cell.
Conclusion
The movement of ions is a foundational process for neuron function, controlled by gradients and specialized channels. Understanding this movement reveals how neurons maintain balance and transmit signals.
Key Takeaways
- The phospholipid bilayer acts as a barrier, with ion channels allowing selective movement of charged particles
- Ion movement across the neuronal membrane is controlled by embedded ion channels, which regulate permeability
- Concentration gradients drive ions from high to low concentration, while electrical gradients drive ions toward opposite charges
- The combined effect of concentration and electrical gradients creates an electrochemical gradient, guiding ion flow
- At equilibrium, ion flow persists through open channels, but there is no net movement
Test Yourself!
- Explain how chemical and electrical gradients affect ion flow.
- Explain ion movement at equilibrium.
Video Lecture