10 Neurotransmitter Release
When an action potential reaches the presynaptic terminal, it triggers the release of neurotransmitters into the synaptic cleft. This process involves the opening of voltage-gated calcium channels, calcium-dependent activation of synaptic proteins, and fusion of synaptic vesicles with the terminal membrane. These events ensure precise and rapid communication between neurons.
Action Potential
As we have covered, when an action potential propagates down the axon to the presynaptic terminal, the electrical signal will result in a release of chemical neurotransmitters that will communicate with the postsynaptic cell.
Animation 10.1. The action potential is a brief but significant change in electrical potential across the membrane. The membrane potential will move from a negative, resting membrane potential, shown here as -65 mV, and will rapidly become positive and then rapidly return to rest during an action potential. The action potential moves down the axon beginning at the axon hillock. When it reaches the synaptic terminal, it causes the release of chemical neurotransmitter. ‘Action Potential Propagation’ by Casey Henley is licensed under a Creative Commons Attribution Non-Commercial Share-Alike (CC-BY-NC-SA) 4.0 International License. View static image of animation
Ion flow in Terminal
Depolarization of the terminal causes voltage-gated calcium channels to open
When the action potential reaches the terminal, there is an influx of sodium ions, just like when the action potential moves down the axon. This inward current causes a depolarization of the terminal, and that depolarization activates voltage-gated calcium channels. There is a strong electrochemical gradient that moves calcium into the terminal.
Animation 10.2. An action potential causes an influx of sodium in the terminal. The depolarization opens voltage-gated calcium channels, and calcium ions flow into the terminal down their electrochemical gradient. The blue, dotted channels represent voltage-gated sodium channels, and the purple, striped channels represent voltage-gated calcium channels. ‘Terminal Calcium Influx’ by Casey Henley is licensed under a Creative Commons Attribution Non-Commercial Share-Alike (CC-BY-NC-SA) 4.0 International License. View static image of animation.
Active Zones
The voltage-gated calcium channels are concentrated in the presynaptic terminal at active zones, the regions of the membrane where small molecule neurotransmitters are released. At active zones, some synaptic vesicles are docked and are ready for immediate release upon arrival of the action potential. Other neurotransmitter-filled vesicles remain in a reserve pool outside of the active zone.
Vesicles filled with neuropeptides do not dock at active zones. They are located outside of the active zone, further away from the membrane and the high density of voltage-gated calcium channels and are therefore slower to release than the small molecule transmitters.
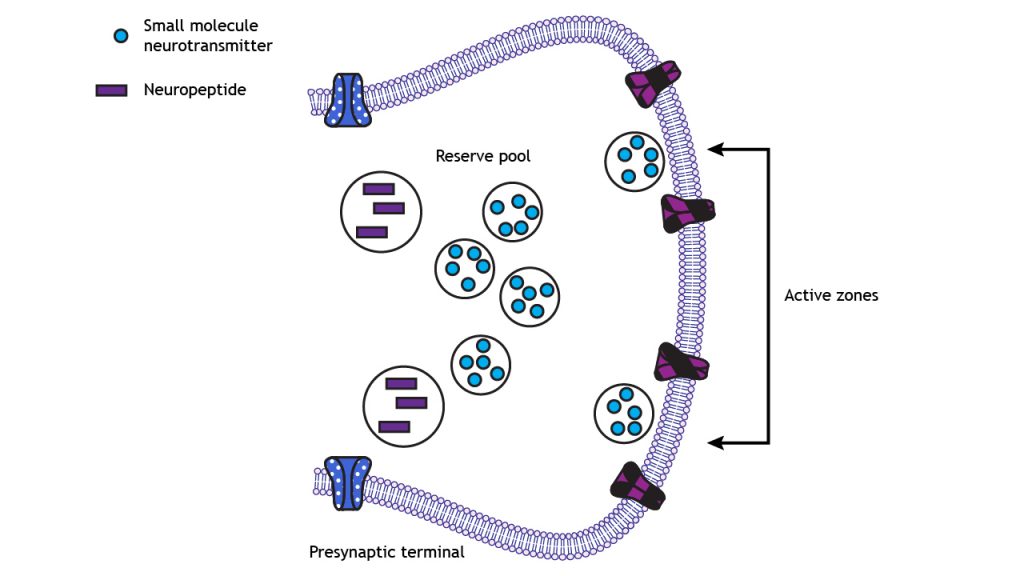
Vesicle Docking
Docking of synaptic vesicles packaged with small molecule neurotransmitters occurs through the interaction of three membrane-bound proteins called SNARE proteins. Synaptobrevin is called a v-SNARE because it is located on the Vesicular membrane. Syntaxin and SNAP-25 are called t-SNARES because they are located on the terminal membrane, which is the Target membrane. The interaction of these three proteins leads to vesicle docking at the active zone.
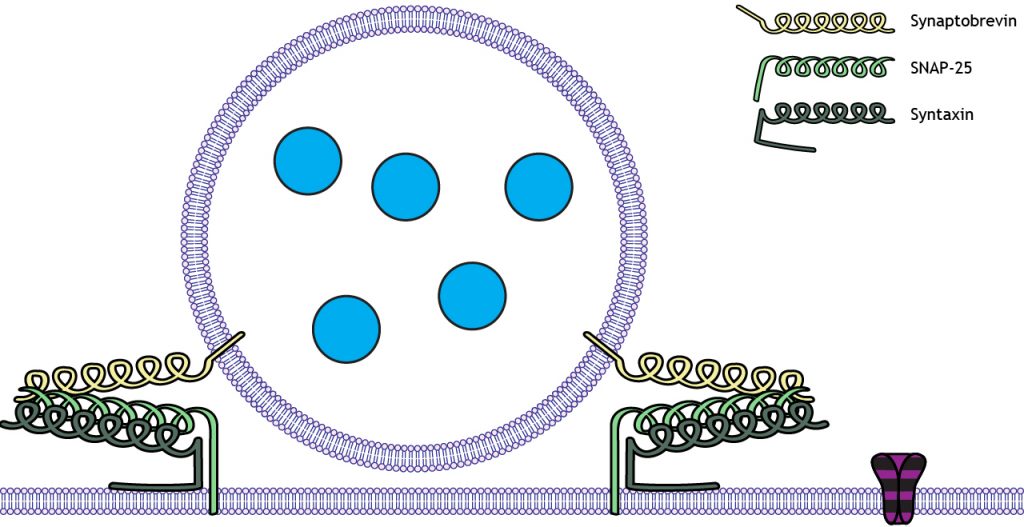
Exocytosis
Exocytosis of neurotransmitters is dependent on calcium
The influx of calcium through the voltage-gated calcium channels initiates the exocytosis process that leads to neurotransmitter release. Calcium enters the cell and interacts with another vesicle-bound protein called synaptotagmin. This protein is a calcium sensor, and when calcium is present at the active zone, synaptotagmin interacts with the SNARE proteins. This is the first step toward exocytosis of the synaptic vesicle.
Animation 10.3. Calcium enters the cell when the voltage-gated channels open. In the presence of calcium, synaptotagmin, a protein bound to the vesicular membrane interacts with the SNARE proteins. The purple, striped channels represent voltage-gated calcium channels. ‘Synaptotagmin’ by Casey Henley is licensed under a Creative Commons Attribution Non-Commercial Share-Alike (CC-BY-NC-SA) 4.0 International License. View static image of animation
Once synaptotagmin interacts with the SNARE proteins, the synaptic vesicle membrane fuses with the presynaptic terminal membrane, exocytosis occurs, and the neurotransmitters released.
Animation 10.4. Once the synaptotagmin-SNARE protein complex forms, the synaptic vesicle membrane fuses with the terminal membrane, and the neurotransmitters are released into the synaptic cleft through exocytosis. The purple, striped channels represent voltage-gated calcium channels. ‘Transmitter Exocytosis’ by Casey Henley is licensed under a Creative Commons Attribution Non-Commercial Share-Alike (CC-BY-NC-SA) 4.0 International License. View static image of animation.
Neurotransmitter Action
After exocytosis of the transmitter molecules, they enter the synaptic cleft and bind to receptors on the postsynaptic membrane. Receptors fall into two main categories: ligand-gated channels and G-protein coupled receptors. The next two chapters cover these receptors.
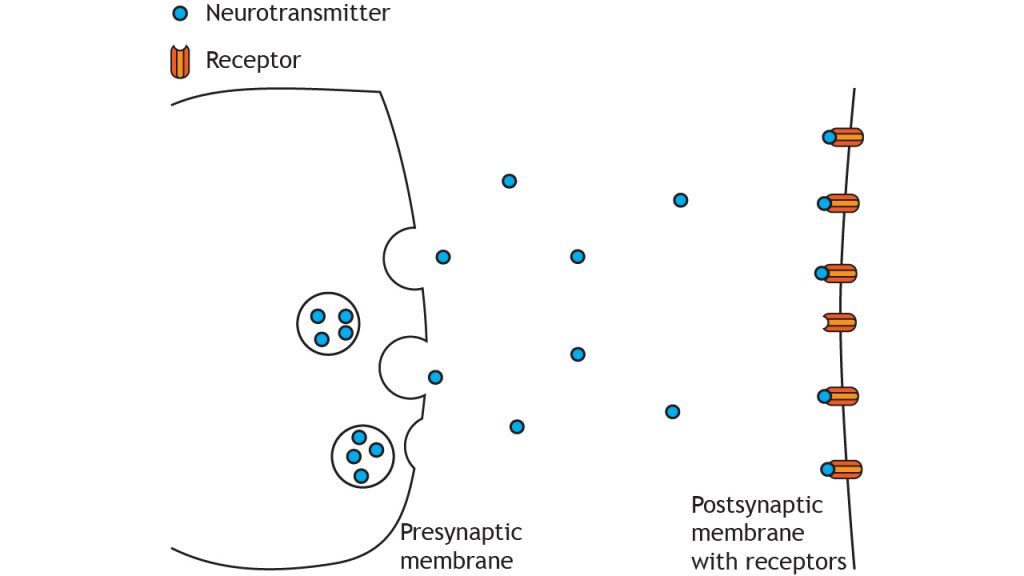
Conclusion
The release of neurotransmitters is a highly coordinated process involving electrical, chemical, and molecular mechanisms. From calcium influx to vesicle fusion, each step ensures efficient signal transmission, laying the foundation for complex neural communication.
Key Takeaways
- Action potentials trigger neurotransmitter release by depolarizing the presynaptic terminal and opening voltage-gated calcium channels.
- Small molecule neurotransmitters dock at active zones on the presynaptic membrane, while neuropeptides are released more slowly from vesicles located outside active zones.
- SNARE proteins (synaptobrevin, SNAP-25, and syntaxin) enable vesicle docking, and synaptotagmin acts as a calcium sensor to initiate exocytosis.
- Neurotransmitters are released into the synaptic cleft via exocytosis and bind to receptors on the postsynaptic membrane.
Test Yourself!
- Describe the events that occur in the presynaptic terminal when an action potential arrives. Include the role of Ca2+.