16 Protein Structure and Function
Andrea Bierema
Learning Objectives
Students will be able to:
- Explain how proteins result in an organism’s traits.
- Explain the relationship between amino acids and proteins.
- Identify examples of proteins.
- Recognize that molecular structure determines molecular interactions and relates to the cellular functions of proteins.
- Describe how protein structure influences its function.
- Describe the relationship between mutation and evolution.
Overview
This chapter is titled “protein structure and function” because protein structure heavily influences its function. The structure of a protein is caused by the chemical properties of its amino acids, which are coded by a DNA sequence (a gene).
This figure illustrates the insulin protein: part of its DNA sequence, part of its amino acid sequence, a representation of the protein, what the protein does, and the trait it causes. Hover over each image to learn more.
Traits
A trait is a specific characteristic of an organism, such as eye color or blood type. Traits can be determined by genes or the environment, or more commonly by interactions between them. The genetic contribution (i.e., the DNA) to a trait is called the genotype. The outward expression of the genotype, including visible and physiological traits, is called the phenotype.
Proteins
Proteins are coded and regulated by genes. These proteins, along with the environment, cause an organism’s traits.
Proteins are one of the most abundant organic molecules in living systems and have the most diverse range of functions of all macromolecules. Proteins may be structural, regulatory, contractile, or protective. They may serve in transport, storage, or membranes; or they may be toxins or enzymes. Each cell in a living system may contain thousands of proteins, each with a unique function. Their structures, like their functions, vary greatly. They are all, however, amino acid polymers arranged in a linear sequence (also referred to as a “peptide”).
Protein types and functions:
Monomers and Polymers
Monomers are molecules that can bind into long chains—these long chains are called “polymers.” In other words, a polymer (“poly” = many) is made of monomers (“mono” meaning “one”).
Amino acids are the monomers that comprise polypeptides (polypeptides being the polymers). A polypeptide folds into a 3D structure called a protein. Scientists use the name “amino acid” because these acids contain both an amino group and a carboxyl-acid group in their basic structure. As we mentioned, there are 20 common amino acids present in proteins. Nine of these are essential amino acids in humans because the human body cannot produce them and we obtain them from our diet. Below are two illustrations depicting the relationship between amino acids and polypeptides.
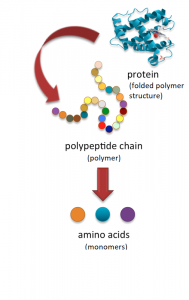
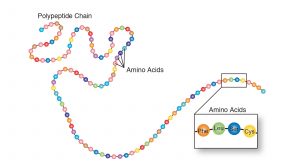
Protein Structure
As mentioned above, a protein’s shape is critical to its function. For example, an enzyme can bind to a specific substrate at an active site. If this active site is altered because of local changes or changes in overall protein structure, the enzyme may be unable to bind to the substrate. To understand how the protein gets its final shape or conformation, we need to understand the four levels of protein structure: primary, secondary, tertiary, and quaternary. See the image below and click on the information hotspots (labeled with an “i”) for explanations.
As seen in the image above, a strand of amino acids folds on itself, creating a unique shape in the tertiary structure of the protein. This is caused by the chemical properties of the amino acids. The chemical properties of the amino acids determine how this shape occurs. For instance, each amino acid is negatively (-), positively (+), or neutrally (N) charged. Negatively charged amino acids bind with positively charged amino acids (neutrally charged amino acids are not affected). Also, the amino acid called cysteine contains sulfur, and sulfurs easily bind with each other, creating a “disulfide bond.” Because of this, cysteines bind with other cysteines. See the table below for a list of all 20 amino acids and their charges. There are other properties that also influence a protein’s shape, such as the amino acid’s polarity. Note that these bonds are not as strong as what is created between amino acids when an amino acid chain is created, but these bonds are strong enough to hold the shape of the protein.
Amino Acid Characteristics
Amino Acid | 3-Letter Abbrev. | 1-Letter Abbrev. | Charge | Disulfide Bond Formation? |
---|---|---|---|---|
Alanine | Ala | A | Neutral | |
Arginine | Arg | R | Positive (+) | |
Asparagine | Asn | N | Neutral | |
Aspartate (Aspartic acid) | Asp | D | Negative (-) | |
Cysteine | Cys | C | Neutral | Yes |
Glutamine | Gln | Q | Neutral | |
Glutamate (Glutamic acid) | Glu | E | Negative (-) | |
Glycine | Gly | G | Neutral | |
Histidine | His | H | Positive (+) | |
Isoleucine | Ile | I | Neutral | |
Leucine | Leu | L | Neutral | |
Lysine | Lys | K | Positive (+) | |
Methionine | Met | M | Neutral | |
Phenylalanine | Phe | F | Neutral | |
Proline | Pro | P | Neutral | |
Serine | Ser | S | Neutral | |
Threonine | Thr | T | Neutral | |
Tryptophan | Trp | W | Neutral | |
Tyrosine | Tyr | Y | Neutral | |
Valine | Val | V | Neutral |
A list of the 20 amino acids common in all living things. The table includes the full name and abbreviations of each amino acid as well as their charge (positive, negative, or neutral). It is also noted which one can create a disulfide bond.
Exercise
Use the chart above to determine which amino acids may bond together to form the tertiary structure.
Example
Here is an example of a polypeptide model depicting how charges influence the tertiary structure. The first and second images are the same, except the second image has hotspots with additional information marked with a question mark (?). The key at the bottom of the image is necessary for interpreting the image.
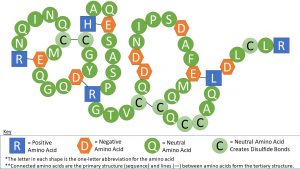
Mutations
Mutations can impact protein synthesis and amino acid sequence. If these mutations are heritable, then they may influence the evolution of a species. Therefore, this chapter includes information on mutations and evolution.
What Are Mutations?
A mutation is a change in DNA, the hereditary material of life. An organism’s DNA codes for the production of proteins, which affects how it looks, how it behaves, and its physiology—all aspects of its life. So, a change in an organism’s DNA can cause changes in all aspects of its life.
The gene encoding the protein ultimately determines the unique sequence for every protein. A change in the nucleotide sequence of the gene’s coding region may lead to adding a different amino acid to the growing polypeptide chain, causing a change in protein structure and function. In sickle cell anemia, the hemoglobin β chain has a single amino acid substitution, causing a change in protein structure and function. Specifically, valine in the β chain substitutes the amino acid glutamic. What is most remarkable to consider is that a hemoglobin molecule is comprised of two alpha and two beta chains that each consist of about 150 amino acids. The molecule, therefore, has about 600 amino acids. The structural difference between a normal hemoglobin molecule and a sickle cell molecule—which dramatically decreases life expectancy—is a single amino acid out of 600. What is even more remarkable is that three nucleotides each encode those 600 amino acids and a single base change (point mutation)—1 in 1800 bases—causes the mutation.
This change to one amino acid in the chain causes hemoglobin molecules to form long fibers that distort the biconcave, or disc-shaped, red blood cells, and causes them to assume a crescent, or “sickle,” shape that clogs blood vessels. This can lead to a myriad of serious health problems such as breathlessness, dizziness, headaches, and abdominal pain for those affected by this disease.
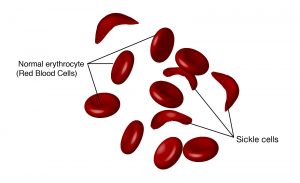
The Causes of Mutations
Mutations happen for several reasons:
- DNA fails to copy accurately: Most of the mutations that we think matter to evolution are “naturally occurring.” For example, when a cell divides, it makes a copy of its DNA and sometimes that copy is not quite perfect. That small difference from the original DNA sequence is a mutation.
Mutations can occur during DNA replication. This image illustrates a double-stranded DNA molecule, opened halfway through. Replication is represented by the partial, red strands connected to the long, blue strands. The inside of the strands has skinny rectangular protrusions representing individual bases. In the “mutant copy,” the wrong color or base has been inserted, which is called a mutation.
- External influences can create mutations: Mutations can also be caused by exposure to specific chemicals or radiation. These agents cause the DNA to break down. This is not necessarily unnatural—even in the most isolated and pristine environments, DNA breaks down. Nevertheless, when the cell repairs the DNA, it might not do a perfect job of the repair. So, the cell would end up with DNA slightly different than the original DNA and hence, a mutation.
Evolution
Biological evolution, simply put, is descent with modification. This definition encompasses small-scale evolution (changes in gene—or, more precisely and technically, allele—frequency in a population from one generation to the next) and large-scale evolution (the descent of different species from a common ancestor over many generations). Evolution is responsible for both the remarkable similarities we see across all life and the amazing diversity of that life, but how does it work?
For evolutionary mechanisms (such as natural selection) to act, there needs to be genetic variation and mutations, or changes, in the DNA. DNA codes for proteins, and when those proteins are produced, mutations create variation. Mutations can be beneficial, neutral, or harmful for the organism, but mutations do not “try” to supply what the organism “needs.” In this respect, mutations are random—whether a particular mutation happens or not is unrelated to how useful that mutation would be.
Because all cells in our body contain DNA, there are lots of places for mutations to occur; however, not all mutations matter for evolution. Somatic mutations occur in non-reproductive cells and won’t be passed on to offspring. Mutations can also be caused by exposure to specific chemicals or radiation. These agents cause the DNA to break down. This is not necessarily unnatural—even in the most isolated and pristine environments, DNA breaks down. Nevertheless, when the cell repairs the DNA, it might not do a perfect job of the repair. So the cell would end up with DNA slightly different than the original DNA and hence, a mutation.
A single germline mutation can have a range of effects:
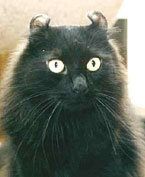
- No change occurs in phenotype: Some mutations don’t have any noticeable effect on the phenotype of an organism. This can happen in many situations: perhaps the mutation occurs in a stretch of DNA with no function, or perhaps the mutation occurs in a protein-coding region but ends up not affecting the amino acid sequence of the protein.
- Small change occurs in phenotype: A single mutation caused this cat’s ears to curl backward slightly.
- Big change occurs in phenotype: Some really important phenotypic changes, like DDT resistance in insects, are sometimes caused by single mutations. A single mutation can also have strong negative effects on the organism. Mutations that cause the death of an organism are called “lethals”—and it doesn’t get more negative than that.
There are some sorts of changes that a single mutation, or even a lot of mutations, could not cause. Neither mutations nor wishful thinking will make pigs have wings; only pop culture could have created the Teenage Mutant Ninja Turtles—mutations could not have done it.
See the “Evolution” chapter in this textbook for more information.
Attributions
This chapter is a modified derivative of the following articles:
“Biological Molecules” by OpenStax College, Biology, CC BY 4.0. Download the original article for free at https://openstax.org/books/biology-2e/pages/3-4-proteins
“Trait” by National Human Genome Research Institute, National Institutes of Health, Talking Glossary of Genetic Terms.
Understanding Evolution. 2020. University of California Museum of Paleontology. 16 July 2020 <http://evolution.berkeley.edu/>. Published with permission.